All optical implementation of scalable quantum information processing: the quantum optical frequency combs.
All optical implementation of scalable quantum information processing: the quantum optical frequency combs.
The last 20 years have seen a rapid development of ideas and proof-of-principle experiments regarding quantum technologies: quantum communication, quantum computation, quantum metrology and quantum simulation. If it is already clear that in principle the quantum world can provide useful advantages in terms of security, efficiency, speed, and sensitivity, we still need to solve the scalability problem. Indeed, generating simple systems for coding and manipulating quantum information is relatively easy. However, it is still not evident how to handle large quantum systems involving many degrees of freedom, in order, for instance, to implement a complete architecture where the quantum approach provides a real advantage compared to conventional systems.
The Quantum Optics group at Laboratoire Kastler Brossel developed in the last years an all-optical solution using the different wavelengths of light as quantum channels and, within each quantum channel, the optical field quadratures to encode quantum information. In this regime, we demonstrated the scalability of multimode entanglement[1,2]. Furthermore, this resource can be tuned at the measurement stage to allow for the on-demand construction of quantum networks suitable for measurement based quantum computing[3,4].
This highly scalable entanglement is of Gaussian nature, in the sense that all measured quantities display Gaussian statistics. While this is sufficient for quantum metrology, it can be proved that any calculation performed using these states can be efficiently simulated using a classical computer. Thus, moving away from Gaussian statistics is a requirement to demonstrate quantum supremacy. We have implemented this de-gaussification operation using mode dependent photon subtraction[5], while demonstrated theoretically that it can lead to a type of entanglement fundamentally different from the Gaussian one[6].
Internship project
The internship will aim at characterizing spectrally broaden femtosecond pulses and use them to perform quantum measurements demonstrating large multimode entanglement compatible with measurement based quantum computing protocols.
In the current experimental setup, the intrinsic number of modes are involved in the generated network could be of the order of 50. All measurements we have implemented so far are able to address a maximum of 16 modes. These are performed using multimode homodyne detection, which is based on the interferences between the signal beam and an intense local oscillator. The oscillator originates from the ultrafast laser source, whose spectral extension is not large enough to efficiently interact with all the generated quantum modes.
We have recently implemented spectral broadening of the local oscillator using self-phase modulation in a short photonic crystal (PC) fiber, expanding the spectrum of the original laser by a factor of two. This source will be characterized, improved, and used for multimode measurement to demonstrated unprecedented number of quantum channels simultaneously available.
PhD project
The internship can be naturally followed by a PhD project that will be based on this experimental setup. The main objectives are to, on the one hand, explore the possibilities of highly multimode non-Gaussian light, a largely unknown regime so far both theoretically and experimentally, and on the other hand to implement non-Gaussian quantum protocols, as for instance quantum verification. This last part could be performed within the collaboration of the group with ATOS Company, in the quest of the future quantum computing architecture.
[1] J. Roslund, R. M. De Araujo, S. Jiang, and C. Fabre, Nature Photonics 8, 109 (2014).
[2] S. Gerke, J. Sperling, W. Vogel, Y. Cai, J. Roslund, N. Treps, and C. Fabre, Phys Rev Lett 114, 050501 (2015).
[3] Y. Cai, J. Roslund, G. Ferrini, F. Arzani, X. Xu, C. Fabre, and N. Treps, Nat Comms 8, 15645 (2017).
[4] G. Ferrini, J. Roslund, F. Arzani, C. Fabre, and N. Treps, Phys Rev A 94, 062332 (2016).
[5] Y.-S. Ra, C. Jacquard, A. Dufour, C. Fabre, and N. Treps, Phys. Rev. X 7, 031012 (2017).
[6] M. Walschaers, C. Fabre, V. Parigi, and N. Treps, Entanglement and Wigner function negativity of multimode non-Gaussian states, arXiv quant-ph, (2017).
Interested candidates should contact Nicolas Treps (nicolas.treps@lkb.upmc.fr), Claude Fabre (claude.fabre@lkb.upmc.fr) or Valentina Parigi (valentina.parigi@lkb.upmc.fr)
For more details, please check our website: www.quantumoptics.fr.
Experimental internship.
Duration: 3 to 6 months. Possible PhD.
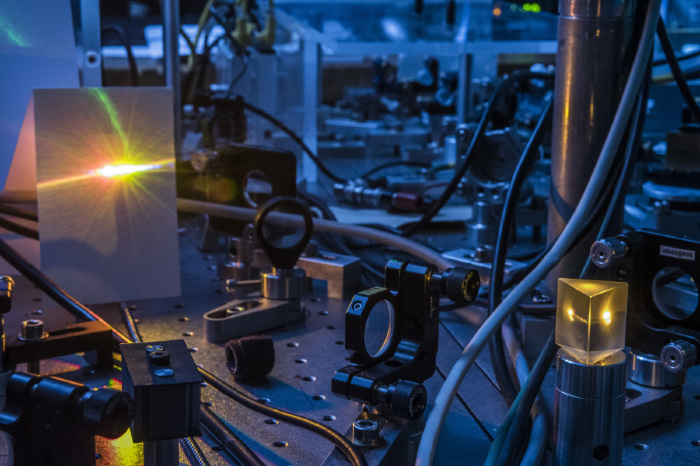