Recent publications
♦ Long-lived circular Rydberg of laser-cooled rubidium atoms in a cryostat
T. Cantat-Moltrecht, R. Cortiñas, B. Ravon, P. Méhaignerie, S. Haroche, J.M. Raimond, M. Favier, M. Brune and C. Sayrin
Phys. Rev. Research 2, 022032 (2020)
♦ Laser Trapping of circular Rydberg atoms
R. Cortiñas, M. Favier, B. Ravon, P. Méhaignerie, Y. Machu, J.M. Raimond, C. Sayrin and M. Brune
Phys. Rev. Lett. 124, 123201 (2020) (arXiv link)
PEOPLE
Permanents: Clément Sayrin, Michel Brune, Jean-Michel Raimond, Serge Haroche
PhDs: Paul Méhaignerie, Yohann Machu, Andrés Durán Hernández, Gautier Creutzer
Postdoc: Maxime Favier (until 2021)
Intern: Aurore-Alice Young (since 04/2023)
Quantum simulation with circular Rydberg atoms
Rydberg atoms are particularly well suited to quantum simulation of interacting spins. Atoms that are excited to these high-principal-quantum-number states interact strongly through dipole-dipole or van der Waals interactions. In our group, we aim at the realisation of a quantum simulator that exploits the unique properties of circular Rydberg atoms, i.e. Rydberg levels with maximal orbital momentum. In a cold environment, these levels live several tens of milliseconds, while the laser-accessible, low-orbital-momentum Rydberg levels live a few 100µs only. Moreover, they can be laser-trapped in light-intensity minima without affecting their lifetime (the circular levels are immune to photoionisation).
In our experiment, we laser-trapped Rubidium-87 atoms in an array of optical tweezers, prepared in a liquid He-4 cryostat. We excite the atoms to circular Rydberg levels and, then, laser-trap them in an array of hollow optical beams. Thanks to the laser trap, and given the strong interactions between circular Rydberg atoms and their long lifetime in the cryogenic environment, the array of interacting atoms is left unaffected over many interaction cycles. This should enable, e.g., the study of the dynamics of condensed-matter systems over long times, their response to quenches or their thermalisation properties, phenomena that are beyond the reach of numerical simulations.
LATEST NEWS
EVENTS
Rodrigo’s PhD Defence
05/05/2020
Rodrigo has successfully defended his PhD thesis entitled Laser trapping of cold circular Rydberg atoms for quantum simulation in front of a jury composed of Jean-Marc Berroir, Isabelle Bouchoule, Frédéric Merkt, Mazyar Mirrahimi and Guido Pupillo, and his supervisors Michel Brune and Clément Sayrin.
This was a time of confinement, and the defence has to go fully online. We could unfortunately only celebrate at distance. We wish him all the best for his future research activities!
Here are glimpses of this peculiar but important event of our team!
Tigrane’s PhD Defence
11/01/2018
Tigrane has successfully defended his PhD thesis entitled Interacting Rydberg Atoms : from Dense Clouds of Rydberg Atoms to Quantum Simulation with Circular Atoms in front of a jury composed of Robin Kaiser, Leticia Tarruell, Jérôme Tignon and Matthias Weidemüller, and his supervisors Michel Brune and Clément Sayrin.
We wish him all the best for his future research activities!
Here are some pictures of this important event for the team!
RESEARCH
Long-lived circular Rydberg states of laser-cooled atoms in a cryostat

Publications
♦ Laser Trapping of Circular Rydberg Atoms
R. Cortiñas, M. Favier, B. Ravon, P. Méhaignerie, Y. Machu, J.-M. Raimond, C. Sayrin and M. Brune
Phys. Rev. Lett. 124, 123201 (2020)
Supplementary Materials
Laser trapping of circular Rydberg atoms
We demonstrate in this work the first laser-trapping of circular Rydberg atoms. Circular Rydberg atoms are prepared from a gas of laser-cooled Rubidium-87 atoms in a cryogenic environment. The atoms are excited within a hollow Laguerre-Gaussian beam prepared with a spatial light modulator. The hollow beam acts as a two-dimensional repulsive barrier for the Rydberg atoms thanks to the induced ponderomotive shift. We observe the inhibition of the thermal cloud (T = 10µK) expansion in the trap, in the direction orthogonal to the beam propagation direction. We observe no loss from the trap within the 10ms of the experiment and no effect on the circular Rydberg lifetime and coherence time. We also induce oscillations of the Rydberg atoms in the trap and measure the trap frequency.
Our method is very general and opens many opportunities for quantum technologies with Rydberg atoms. The 10 ms trapping time corresponds to thousands of interaction cycles in a circular-state-based quantum simulator. It is also promising for quantum metrology and quantum information with Rydberg atoms, by bringing atom-field interaction times into unprecedented regimes.
Long-lived circular Rydberg states of laser-cooled atoms in a cryostat
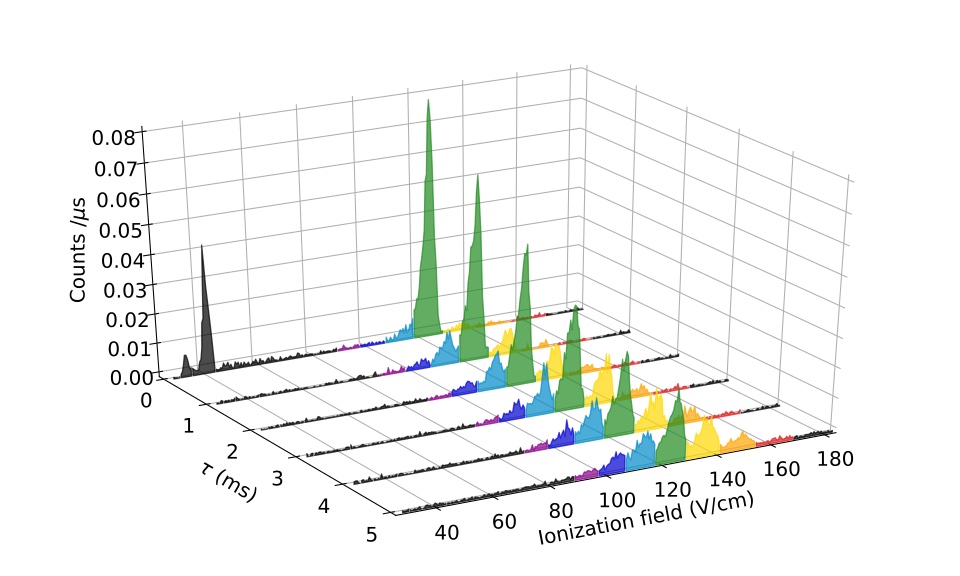
Publications
♦ Long-lived circular Rydberg states of laser-cooled rubidium atoms in a cryostat
T. Cantat-Moltrecht, R. Cortiñas, B. Ravon, P. Méhaignerie, S. Haroche, J.-M. Raimond, M. Favier, M. Brune and C. Sayrin
Phys. Rev. Research 2, 022032 (2020)
Supplementary Materials
Long-lived circular Rydberg states of laser-cooled atoms in a cryostat
The exquisite properties of circular Rydberg atoms make them particularly appealing for emergent quantum technologies. However, to benefit from their long natural lifetimes, of a few 10ms, requires laser-cooled atoms and a cryogenic environment reducing the blackbody radiation temperature. At room temperature, microwave blackbody radiation induced transfers limit the lifetime of the circular levels to a few 100µs.
In this work, we demonstrate the preparation of circular Rydberg levels from a gas of laser-cooled atoms in a cryostat. Rubidium-87 atoms are laser-cooled in a mirror magneto-optical trap and further cooled-down to 10µK in optical molasses. We then excite them to the circular Rydberg level with a principal quantum number of n=52 and found his lifetime to be 3.7 ms. By monitoring the transfers between adjacent circular levels, we estimate in situ the microwave BBR temperature to be (11 ± 2) K. The measured atomic coherence time (270 μs) is limited here only by technical magnetic field fluctuations. This work opens interesting perspectives for quantum simulation and sensing with cold circular Rydberg atoms.
Towards quantum simulation with circular Rydberg atoms
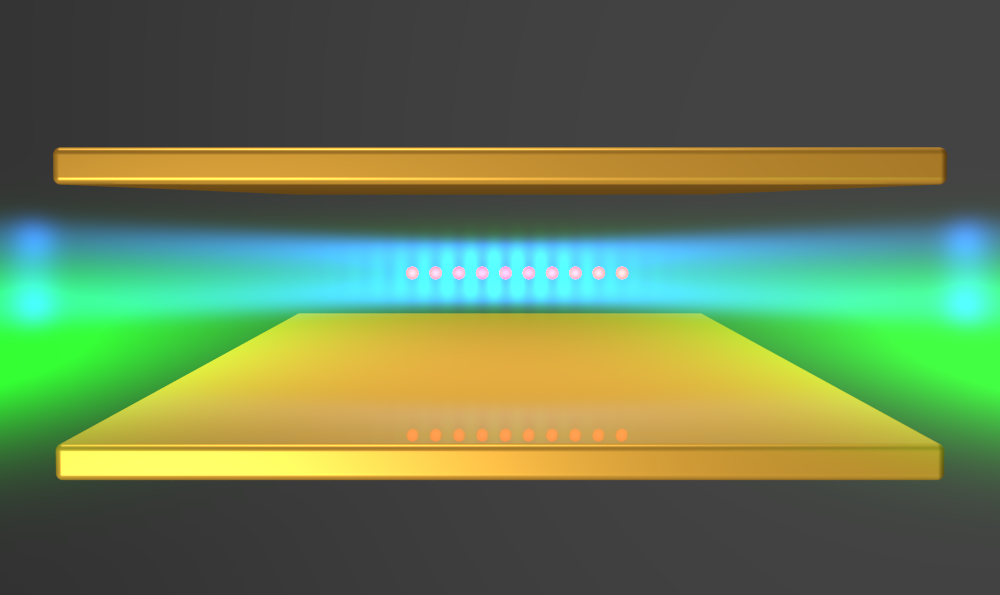
Publications
♦ Towards quantum simulation with circular Rydberg atoms
T.L. Nguyen, J.-M. Raimond, C. Sayrin, R. Cortinas, T. Cantat-Moltrecht, F. Assemat, I. Dotsenko, S. Gleyzes, S. Haroche, G. Roux, T. Jolicoeur, M. Brune
Phys. Rev. X 8, 011032 (2018)
Supplementary Materials
Towards quantum simulation with circular Rydberg atoms
The main objective of quantum simulation is an in-depth understanding of many-body physics. It is important for fundamental issues (quantum phase transitions, transport, . . . ) and for the development of innovative materials. Analytic approaches to many-body systems are limited and the huge size of their Hilbert space makes numerical simulations on classical computers intractable. A quantum simulator avoids these limitations by transcribing the system of interest into another, with the same dynamics but with interaction parameters under control and with experimental access to all relevant observables. Quantum simulation of spin systems is being explored with a large variety of experimental systems. Some of the most advanced ones rely on trapped ion systems, superconducting circuits, trapped ultra-cold ground state atoms or even atoms excited to low angular momentum Rydberg levels.
We propose a new paradigm for quantum simulation of spin-1/2 arrays providing unprecedented flexibility and allowing one to explore domains beyond the reach of other platforms. It is based on laser-trapped circular Rydberg atoms.
The intrinsic lifetime of circular Rydberg atoms lies in the ms-range. This very long lifetime stems from the fact that a circular Rydberg atoms \( |nC\rangle\) can only decay to the \( |(n-1)C\rangle\) circular Rydberg level by the emission of a microwave photon of wavelength \(\lambda\). By placing a circular Rydberg atom in a plane-parallel condenser of size \(d\leq\lambda/2\), spontaneous emission can be efficiently inhibited and the lifetime of the circular level increased by several orders of magnitude.
In addition, while photoionisation is a severe limitation for laser trapping of low-angular momentum Rydberg levels, circular Rydberg levels do not photoionise. The combination of a Laguerre-Gaussian beam (lateral confinement) and of two crossed Gaussian beams (longitudinal confinement) then realise a very efficient trap for the low-field seeking circular Rydberg levels. Trapping lifetimes in the minute range are realistic with state-of-the-art techniques.
Finally, using an innovative approach, namely a van der Waals variant of the evaporative cooling method, ultra-cold defect-free circular atom chains containing several tens of atoms can be prepared. A chain of about 40 atoms can be deterministically prepared using this method. Its lifetime is on the range of seconds! This method also leads to the individual detection of arbitrary spin observables and, thus, to the measurement of spin-spin correlation functions.
The proposed simulator realises an XXZ spin-1/2 Hamiltonian with nearest-neighbor couplings ranging from a few to tens of kHz. The simulated Hamiltonian takes the general form
\( H/h = \frac{\Delta}{2}\sum_{i=1}^N \sigma_i^z + \frac{\Omega_0}{2}\sum_{i=1}^N \sigma_i^x + J_z \sum_{i=1}^{N-1} \sigma_i^z \sigma_{i+1}^z + J \sum_{i=1}^{N-1} \sigma_i^x \sigma_{i+1}^x + \sigma_i^y \sigma_{i+1}^y. \)
All the model parameters can be tuned at will, making a large range of simulations accessible. In particular, by a mere tuning of an electric and a magnetic field, the ratio \(J_z/J\) can be tuned from -3 to 3. The accessible phase diagram is extremely rich. The typical interaction time, \(1/4J\), is on the order of \(15\mu\mathrm{s}\). The system evolution can thus be followed over \(10^4\) to \(10^5\) interaction cycles, long enough to be relevant for ground-state adiabatic preparation and for the study of thermalization, disorder or Floquet time crystals. This platform presents unrivaled features for quantum simulation.
Dipole blockade and microwave spectroscopy

Publications
♦ Microwaves Probe Dipole Blockade and van der Waals Forces in a Cold Rydberg Gas
R. Teixeira, C. Hermann-Avigliano, T.-L. Nguyen, T. Cantat-Moltrecht, J.-M. Raimond, S. Haroche, S. Gleyzes, M. Brune
Phys. Rev. Lett. 115, 013001 (2015)
Supplementary Materials
Dipole blockade and microwave spectroscopy
Because of their strong electric polarizability, Rydberg atoms interact very strongly through dipole-dipole interaction. During a resonant laser excitation of Rydberg levels in a dense atomic cloud, the strength of the interaction leads to the so-called dipole blockade effect [Lukin et al. (2001)]: every Rydberg atoms is surrounded by a blockade sphere into which no other Rydberg atom can be excited. At saturation, the distance between the Rydberg atoms then corresponds to the radius of this sphere, the so-called blockade radius. However, when the laser excitation is detuned from resonance, the dipole interaction can facilitate the excitation of a Rydberg atom at a distance called facilitation radius from an initial Rydberg seed. The value of the facilitation radius is controlled via the detuning of the excitation light.
In our experiments, we have been recently able to demonstrate that the relative distance between Rydberg atoms excited in a cold gas of atoms, trapped in the vicinity of our atomchip, can be controlled via the detuning of the excitation laser. Using microwave spectroscopy, we have measured directly the interaction energy distribution of the Rydberg gas, from which we can deduce the Rydberg atoms spatial distribution. By recording the time evolution of the interaction energy distribution, we have also observed the dynamics of the Rydberg gas, which expands due to the strong repulsive interaction between the atoms. In particular, we have been able to observe the breakdown of the frozen gas approximation, few µs only after the preparation of the Rydberg gas.
PEOPLE